It ain't magic: “time-reversal” in neutron star collisions
Scientists at the AEI find explanation for X-Ray afterglows after gigantic explosions in space
Neutron star collisions are extreme events: the evidence points towards them being the origin of short gamma-ray bursts, which are among the most luminous explosions in the Universe. The burst is most likely produced when the massive object formed in the collision collapses to a black hole. However, satellites often detect not only the extremely short gamma-ray burst, but also a subsequent strong emission of X-rays, lasting several hours or more, that cannot be explained by the very short activity of the newly-formed black hole. In a recent publication in the Astrophysical Journal Letters AEI scientists propose an explanation for this apparent inconsistency. The explanation involves a time reversal in the production and observation of the gamma-ray and (part of the) X-ray signals. It also opens up new possibilities for multimessenger astronomy.
Short gamma-ray bursts release – in less than two seconds – as much energy as would be obtained by blowing up two million trillion trillion megatonnes of the explosive TNT. The most likely explanation for their origin is the merger of two neutron stars, forming a very massive metastable neutron star, which then collapses into a black hole under its own gravity. This black hole is at first surrounded by a thick disk of matter, which is accreted onto the black hole in about a second. The gamma-ray burst is produced in this accretion process, provided that extremely strong magnetic fields are present.
After decades of research, the exact mechanism behind short gamma-ray bursts is still a mystery. Computer simulations at the AEI are used to investigate this phenomenon. In the last two years, they provided insight, e.g., into how the enormous magnetic fields needed for gamma-ray bursts could form. "We are continually getting better in modeling the conditions needed for the generation of gamma-ray bursts in numerical simulations on supercomputers", says Daniel Siegel, PhD student at the Max Planck Institute for Gravitational Physics (Albert Einstein Institute/AEI), who, together with his colleague Dr. Riccardo Ciolfi (now postdoctoral scholar at the University of Trento), is trying to solve this mystery. "Simulations are an indispensable tool for understanding what is going on. This is because satellite and ground-based observations can only give us indirect information about the events that take place when a short gamma-ray burst occurs." In their latest publication, Ciolfi and Siegel propose a scenario that not only accounts for the gamma-ray burst and the X-ray emission, but also envisages that the X-rays should not only be seen after the burst but also before it – a surprising new prediction.
Apparent time-reversal explains mysterious afterglow
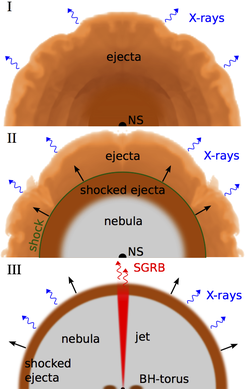
Simulations on one of the AEI's computer clusters showed what happens if a long-lived and extremely dense neutron star is formed in the collision of two neutron stars. At first, in this remnant neutron star matter rotates at different speeds and the star ejects a strong mass-laden wind. This wind is so dense that it is almost impenetrable by radiation, and therefore stores much of the electromagnetic energy which is emitted by the neutron star (Fig. 1, I). Only a small amount of energy is already radiated away in X-rays. After this stage, according to further mathematical modelling, the neutron star becomes a uniformly rotating body and produces a plasma nebula made up of electrons, positrons and photons (Fig. 1, II). The high pressure of the photons in this nebula produces a shockwave in the surrounding ejected matter, accelerating it to relativistic speeds. Rotational energy of the neutron star is transferred to the plasma and stays there, and the loss of this energy eventually causes the neutron star to collapse into a black hole with an accretion disk (Fig1, III). In less than a few seconds, the newly formed black hole accretes the disk and generates a focused jet-like outflow of material, which can easily penetrate the nebula and the surrounding shell of matter and eventually produces the short gamma-ray burst. The main part of the energy extracted from the neutron star before it collapsed into a black hole is still stored in the nebula and the surrounding shell of matter – it is radiated away as X-rays over several hours or more.
It is this delayed radiation of the stored energy that causes the apparent reversal of time when observing the gamma and X-rays: even though rotational energy is given off before the neutron star collapses into a black hole, this energy is stored and most of it is emitted after the gamma-ray burst, caused by the actual collapse. The calculations made by Siegel and Ciolfi show that the delayed emission of energy conforms to observational data and can therefore explain the afterglow that is actually seen. In contrast, the X-rays that are not affected by the time reversal, i.e. those that are emitted before the gamma-rays (Fig. 1, I&II), cannot be observed by satellites yet. Satellites currently use the stronger gamma-ray signal to trigger X-ray observations, since the weaker X-ray signal can only be detected if the sky location is known to some precision.
"Our time reversal does not use magic time travel, but entirely un-magical storage of energy in a medium, from where it is released after a while", says Siegel, and Ciolfi adds: "A real challenge now is for astronomers to observe X-rays before the gamma rays. This would be strong evidence for our type of 'Time reversal' and the scenario which we propose."
New perspectives for astronomy
If gravitational waves and electromagnetic radiation could be observed together, then, for the first time, it would be possible to accurately determine the lifetime of a neutron star (to an accuracy of better than one percent).
Before two neutron stars merge, they circle each other in ever tightening orbits. While this happens, they lose energy through gravitational waves. The strongest gravitational-wave signal is emitted when the actual collision and merger takes place (shortly before the stage illustrated in Fig. 1, I), and this gravitational-wave signal is separated from the short gamma-ray burst (Fig. 1, III) precisely by the time the extremely dense neutron star exists. Gravitational-wave detectors are therefore ideal to warn of imminent short gamma-ray bursts, so that they can be detected by ground or space based telescopes. The new scenario discovered at the AEI opens up fascinating possibilities for multi-messenger astronomy by simultaneously observing electromagnetic and gravitational waves, which will become possible when gravitational-wave detectors like advanced LIGO and Virgo come online this year.