Gravitational waves detected from second pair of colliding black holes
Key contributions from Max Planck and Leibniz Universität Hannover researchers to second gravitational-wave event found in LIGO data
On December 26, 2015 the LIGO instruments detected a second gravitational wave. The observed signal originated from a pair of merging black holes of about 14 and 8 solar masses – smaller than the ones detected on September 14, 2015. Researchers from the Max Planck Institute for Gravitational Physics in Potsdam and Hannover and the Leibniz Universität Hannover have contributed to the discovery in several key areas: the development of highly accurate gravitational-wave models, searches to detect faint signals, assessment of their statistical significance, determining their astrophysical parameters, and advanced detector technology. This second discovery proves that a new era of gravitational-wave astronomy has begun.
Gravitational waves are one of the key predictions of Einstein's general theory of relativity. They were first directly detected on September 14, 2015 by the twin LIGO instruments. The detection of the second gravitational wave on Earth is accepted for publication in Physical Review Letters.
Researchers from the Max Planck Institute for Gravitational Physics (Albert Einstein Institute; AEI) and Leibniz Universität Hannover (LUH) have made important contributions to this detection, having developed highly accurate models for gravitational-wave signals from binary black holes which also include the black holes' spins. These models were essential both to detect the signal and to determine the astrophysical properties of the source. AEI and LUH scientists developed and implemented important elements of the data analysis algorithms and software, provided the most powerful computing systems used in the analysis, and played a central role in proving that the event was real and not a statistical fluke. They have also contributed advanced detector techniques tested at the GEO600 gravitational-wave detector near Hannover.
The second gravitational wave detected on Earth
The signal was detected in LIGO's first observation run “O1” on December 26, 2015 at 3:38:54 UTC by both of the LIGO detectors, and was consequently named GW151226. The wave arrived 1.1 ms earlier at the Livingston detector than at the Hanford detector.
The second observation GW151226 was much weaker than the first detection GW150914 and was buried in the detector noise. Therefore, a so-called “matched-filter” search was essential for the detection. In such searches the data are compared to (or filtered with) many predicted signals (or templates) in order to find the best match. The templates are based on highly accurate gravitational-wave models developed at the AEI, which allowed the LIGO science team to show that the signal was caused by the inspiral and merger of two black holes.
After the initial detection, subsequent matched filtering, for which half of the computing power was provided by the AEI, revealed the astrophysical properties of the binary black hole. It consisted of one black hole with about 14 times the mass of our Sun and one with about 8 solar masses. At least one of them spins on its axis. The black holes merged at a distance of some 1.4 billion light-years from Earth. The merger emitted the equivalent of about 1 solar mass in gravitational wave energy and left behind a rotating 21 solar mass black hole.
The signal, extracted from the detector noise, differs in several key aspects from the first detection. Because of the smaller masses, the signal was registered by the instruments for a longer time (about 1 second) and for about of 27 orbits of the black holes before merger. (For the first detection only the last 5 orbits were observable.) Over this time the gravitational wave increased in frequency from 35 Hz to 430 Hz. The signal's peak strain amplitude of about 3×10-22 made it about three times weaker than the first detection.
Matched-filter analyses and waveform models crucial for detection of faint signal
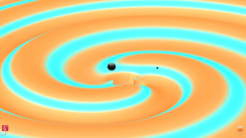
Scientists of the Astrophysical and Cosmological Relativity division at the AEI in Potsdam played leading roles in designing the matched-filter analyses that detected GW151226 within about a minute of its arrival and subsequently achieved a statistical significance greater than 5 standard deviations. Those searches used waveform models for binary black holes that were developed at the AEI in Potsdam and the University of Maryland. Max Planck researchers built follow up analysis methods and employed those waveform models to unveil the astrophysical properties of GW151226 and found that the component black holes were much lighter than the ones in GW150914.
“It's fabulous that our waveform models have pulled out from the noise such a weak but incredibly valuable gravitational-wave signal!” says Alessandra Buonanno, director at the AEI in Potsdam and professor at the University of Maryland. “GW151226 perfectly matches our theoretical predictions for how two black holes move around each other for several tens of orbits and ultimately merge. Remarkably, we could also infer that at least one of the two black holes in the binary was spinning!”
Max Planck scientists within the Simulating eXtreme Spacetime collaboration also ran numerical-relativity simulations of binary black-hole coalescences in General Relativity with parameters close to GW151226. Comparing the results with the waveform models used to infer the astrophysical properties of the source, they found excellent agreement over the entire signal, further verifying that GW151226 was generated by the collision of two stellar-mass black holes in General Relativity.
Analysis methods and computing power
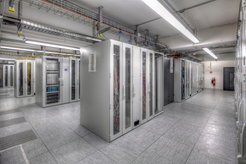
Members of the Observational Relativity and Cosmology division at AEI Hannover developed and implemented many of the algorithms and software used in the analysis of the LIGO observational data. These analyses were used, for example, to establish the statistical significance GW151226 and determine its parameters. In fact about 50% of the data analysis was performed on the Atlas supercomputer operated by the division. Atlas is the most powerful computer cluster in the world designed for gravitational-wave data analysis and has contributed significantly more than any other system used by the LIGO and Virgo Collaborations.
“Now even the skeptics have to admit that our first detection was not a fluke,” says Bruce Allen, Managing Director of the AEI and Honorary Professor at LUH.“I am now totally confident that in the next few years we will detect dozens of similar black hole mergers, and learn a lot about the universe. It is very satisfying to see that the data analysis methods we have invented in the past twenty years work as well as we had hoped.”
Advanced detector techniques from GEO600

The GEO collaboration includes Max Planck and Leibniz Universität researchers together with UK colleagues. They designed and operate the GEO600 gravitational-wave detector near Hannover, Germany. It is used as a think tank and testbed for advanced detector techniques. Most of the key technologies that contributed to the unprecedented sensitivity of Advanced LIGO (aLIGO) and enabled the first discoveries have been developed and tested within the GEO collaboration. Examples of these are signal recycling, resonant sideband extraction, and monolithic mirror suspensions. AEI researchers together with the Laser Zentrum Hannover e.V. also developed, built, and installed the aLIGO high-power laser systems, which are crucial for the high-precision measurements.
“With this second observation we truly are on the path to genuine gravitational-wave astronomy. We can start to explore the variety of sources on the unknown dark side of the Universe,” says Karsten Danzmann, director at the AEI in Hannover and director of the Institute for Gravitational Physics at LUH. “After so many years of research, development, and preparation it is very satisfying to see our vision finally come true.”
Next steps
Advanced LIGO’s next data-taking run “O2” will begin this fall and is expected to last for about six months. By then, further improvements in detector sensitivity should allow LIGO to reach as much as 1.5 to 2 times more of the volume of the universe. The GEO600 gravitational-wave detector will also take part in the observation run. The Virgo detector is expected to join in the latter half of the O2 run.