Tides in binary star systems
When neutron stars emit gravitational waves
Scientists at the Max Planck Institute for Gravitational Physics in Potsdam develop an accurate model for the detection and interpretation of gravitational waves emitted by neutron stars in binary systems. This model contains, for the first time, a realistic description of how neutron stars are deformed just before they collide. As the deformation depends on the exotic physics in neutron star interiors and directly influences the gravitational waves more detailed information about the science contained in the expected signals is now available. This will enable more robust measurements leading to an improved understanding of the properties of the densest objects in our universe.
The first discovery of gravitational waves from merging black holes announced earlier this year has initiated the use of gravitational waves as unique probes of the most violent astrophysical processes. A highly anticipated source of gravitational waves are collisions of neutron stars. Neutron stars are among the most fascinating objects in the universe: they have up to two times the mass of our Sun contained in a diameter of less than 20 kilometers. The nature of such extremely dense matter has remained a mystery for decades. If we could probe the interiors of neutron stars, we could understand the unknown physics of these extreme celestial bodies. Gravitational wave astronomy will allow us to do so, as neutron stars in binaries emit waves in spacetime when they merge. These gravitational waves carry unique information about the neutron stars. However, such signals of astrophysical origin are weak compared to the instrumental noise of current detectors. Nevertheless, the extraction of a signal from the noisy data and its analysis becomes possible with accurate theoretical models of the plausible signals that these systems emit. In particular, the Effective One Body model for binary black holes developed at the Max Planck Institute for Gravitational Physics in Potsdam and the University of Maryland was instrumental in assessing the highest detection confidence and maximizing the science gains from the recent discovery of gravitational waves with LIGO detectors.
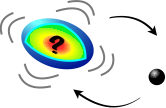
The present work extends this Effective One Body model to include the imprint of the rich neutron star physics on the waves. When a neutron star orbits another compact object - a second neutron star or a black hole - it is deformed due to tidal forces (figure 1). This effect is reminiscent of what happens here on Earth when the moon’s gravity raises the ocean tides. Similarly, the neutron star deforms in response to its companion. This has been the focus of several past studies. The present work significantly improves the modeling of tidal effects by taking into account that internal oscillations in the neutron star will arise when the companion’s tidal force varies at a frequency that is close to a characteristic frequency of the star itself. This is analogous to oscillations of a bridge excited by a band marching at a pace that matches the bridge’s characteristic frequency. The characteristic frequency of neutron stars is in the kHz range and is approached just before the neutron star and its companion merge. In this final stage of the collision the neutron star orbits its companion in less than a millisecond at about half of the speed of light. Both the amount of tidal deformation and the characteristic frequency of a neutron star depend sensitively on the microphysical properties of the neutron star matter. Any tidal response of the star leaves a distinct imprint on the gravitational waves emitted by the binary. Thus, gravitational waves will reveal unique information about the exotic interior of the neutron stars.
“Our detailed model more accurately predicts the waveforms and thus tells us what to look for in the data”, says Dr Andrea Taracchini, co-author of the study and scientist in the Astrophysical and Cosmological Relativity division at AEI. “We tested our model against results from numerical relativity simulations produced by our collaborators in the US and Japan. The model shows a better agreement with the numerical results than models which neglect the characteristic frequency." "This means that our model is capturing genuine physical effects”, says Dr Tanja Hinderer, main author of the paper, scientist at the University of Maryland and long-term visitor at the AEI. Numerical simulations provide the most realistic predictions for gravitational waves, however, they are too expensive to deliver enough waveforms for the detectors. The newly developed analytical model not only enables generating arbitrarily many waveforms, but also explains physical characteristics of the waves.
The search and analysis of gravitational waves requires detailed knowledge of an enormous number of different waveforms. Various different parameter combinations must be calculated: different compositions of the binary system, different mass ratios, spins, and models of neutron star matter. The new analytical model allows to calculate thousands of waveforms in a short time. The extraction of science from the gravitational wave data is then performed using these templates.