On the deceleration behaviour of black holes
Researchers use the concept of “anti-kick” to explain why the speed suddenly decreases after the collision of such exotic objects
Kicking is not only associated with football: if two black holes approach each other so closely as to collide and merge, the resulting black hole recoils and then races through the universe at a speed of up to several thousand kilometres per second. Sometimes, however, it experiences a sudden decrease in speed - a behaviour for which there was no convincing explanation. Scientists from the Max Planck Institute for Gravitational Physics have now found a solution to the puzzle: there is a type of recoil in the opposite direction that reduces the speed of the whole system. In this "anti-kick" the black hole emits gravitational waves to reach its energetically optimum shape: a sphere.
Viewed from the outside, a black hole is not a tangible object but a region in space that draws in matter from its surroundings with great force. The boundary which separates this region from the rest of space is called the horizon. In the simplest case, the horizon is perfectly spherical and floating in space. Anything crossing the horizon from the outside is unable to leave again. Not even light can escape this gravitational trap – hence its name. Black holes are considered to be important components of models which astrophysicists use to explain stellar evolution or the interior of active galactic nuclei.
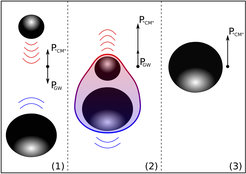
Luciano Rezzolla, head of the ‘Numerical Relativity’ research group at the Max Planck Institute for Gravitational Physics (Albert Einstein Institute, AEI), and his colleagues Rodrigo Macedo and José Luis Jaramillo started by examining a simple system. In this model, a smaller and a large black hole move linearly towards each other and collide head-on. The smaller black hole moves faster, has a high downward momentum and emits strong gravitational waves downwards. Since every action also produces a reaction, the overall system moves upwards - this is the "kick" (left part of Fig. 1). The black hole produced by the merger initially is not spherical, but is deformed and has a type of “bump” at the top (central part of Fig. 1). To smooth this asymmetry and achieve the energetically more favourable spherical shape, more momentum is radiated upwards by means of gravitational waves: this "anti-kick" therefore decelerates the resulting black hole. It still moves upwards, albeit at a reduced speed (right part of Fig. 1).
"This simple model takes us a lot further towards understanding black hole collisions. In our publication we provide an intuitive explanation for a process whose mathematical details are horribly complex," says Luciano Rezzolla. "It is important in physics to understand complex phenomena and provide clear explanations. This is the significance of the research results of Rezzolla and his team," adds Bernard F. Schutz, Director of the Astrophysical Relativity Division.
The generation of a large kick in the merger of black holes has a direct impact in astrophysics: Depending on its size, in fact, it determines the number of galaxies containing supermassive black holes at their centres since black holes can even be kicked out of their galaxies.
The explanation of the anti-kick suggests a methodology to probe the physics around a black hole by monitoring the geometry near its horizon. This approach may help understanding some fundamental aspects of black-hole physics.
Background information
Black holes
The more compact and the more massive an object is, the stronger the gravitational influence it exerts on its direct neighbourhood. Black holes started out as a theoretical idea, predicted by Einstein’s general relativity, an exotic consequence of the way Einstein’s gravity distorts spacetime. Nowadays, however, they represent an important building block of astrophysical models, from stellar evolution over to the active centre of active nuclei of some galaxies.
Black holes themselves are not solid object, but rather a region of space with very special properties: matter or light can enter that region from the outside, but nothing that has entered can ever return get out. The border separating this region from the rest of the world is called the horizon of the black hole. In the simplest case, that is when the black hole is not rotating, the horizon as a perfectly spherical shape, which can however be distorted if the black hole rotates. Nothing really special happens when crossing the horizon but besides not being able to get out again, anything entering a black hole is doomed to encounter the „singularity“ at its centre. This is a region of infinite density and energy, where the laws of classical physics break and which requires a theory of quantum gravity to be properly described.
Cosmic collisions
Collisions of astronomical compact objects such as black holes and neutron stars are among the most powerful events in the universe. Scientists of the numerical relativity group (leader: Professor Luciano Rezzolla) at the Max Planck Institute for Gravitational Physics simulate mergers of black holes and neutron stars, calculate the energy released in these events and comparing it with the one obtained from astronomical observations. In addition, because of their compactness and mass, black holes and neutron stars are the most promising sources of gravitational waves.
Gravitational-wave astronomy
As black holes, also gravitational waves are a direct prediction of Einstein’s theory of general relativity and represent simple ripples in the fabric of space and time which are produced by large and compact masses when moving at very large velocities. Scientists from all over the world anxiously await for the first direct detection of gravitational waves. With gravitational wave astronomy we will have the possibility to know much of the still unknown 96% of the Universe. By detecting them we will effectively “listen” to the Universe, that is, observe it in a range of frequencies which is very different from the at which we routinely obtain astronomical electromagnetic information. Besides dealing with the enormous experimental difficulties related with the detection of the gravitational-wave signals, the search for gravitational waves requires detailed knowledge of the expected signals and cutting-edge methods for managing huge amounts of data.
In the ‘Astrophysical Relativity’ division at the Max Planck Institute for Gravitational Physics (Albert Einstein Institute) in Potsdam-Golm, lead by Professor Bernard F. Schutz, both research fields are investigated in internationally leading working groups.