Measuring the quantum limit with a perfect silicon mirror
Scientists from Hannover and Jena have developed a new method of making a silicon crystal into a perfect mirror: they have etched the surface into a specially structured nano-lattice. Such a surface completely reflects laser light - an effect which until now could only be achieved by vapor deposition with a reflective layer system. This new method is highly promising for high-precision measurements in the fields of quantum mechanics and gravitational wave research. The researchers recently published their results in the scientific journal Physical Review Letters, No. 104.
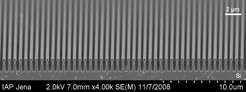
For highly precise experiments, especially in quantum optics and gravitational wave research, optical mirrors are required that reflect light as efficiently as possible. In order to achieve the required high level of reflectivity, the conventional method is to coat a ground crystal or polished quartz glass with numerous layers of materials that differ optically (with a so-called “coating”). However, there is a disadvantage to this method. The coating material manifests a particularly strong Brownian motion (heat movement of the particles in the coating). As a result, when measurements are undertaken, a thermal background noise is superimposed on the actual signal, thereby restricting the accuracy of measurement.
Daniel Friedrich and Frank Brückner, part of the Research Groups of Prof. Roman Schnabel (Institute of Gravitational Physics, QUEST Cluster of Excellence, Leibniz Universität Hannover and the Max Planck Institute for Gravitational Physics, Hannover), and Prof. Andreas Tünnermann (Institute for Applied Physics, Friedrich Schiller University of Jena and Fraunhofer Institute for Applied Optics and Precision Mechanics, Jena), have together developed a new method designed to eliminate disturbing noise. Harnessing this method, they engraved a nano-lattice onto the surface of a silicon crystal. This lattice structure functions as a resonant wave guide for light of a certain wave length, in this case infrared radiation at 1,550 nm. Perpendicularly incident light is diffracted by the lattice geometry into several partial beams, which subsequently become superimposed (“interference”). However, in this particular surface structure, constructive interference only occurs in the reverse direction. Light rays moving in other directions cancel one another out. All in all, this leads to perfect reflection. “This effect is very similar to that encountered in certain butterfly species in which the wings of Morpho butterflies have such a bright blue shimmer, because their flight surface has also been provided with a periodic nano-structure that reflects certain colors of the incident light selectively,” explains Frank Brückner.
The reflectivity achieved in this experiment is exactly 99.8%, but up to 100% is theoretically possible. Smoothly polished, a silicon crystal would only reflect infrared radiation up to 30% in the case of perpendicular incidence. As a result, the nano-lattice replaces the coating with materials that have varying refractive indices.
“By dispensing with optical coatings, the thermal noise associated therewith should also disappear. In regard to measuring processes at the quantum limit, this noise is one of the most important sources of interference and significantly reduces sensitivity of the measurement,” says Daniel Friedrich. “With a nano-lattice structure acting as a mirror on crystal surfaces, we now expect a whole new quality in high-precision measurements conducted in different areas of research.”
The results of their work were published in an article that appeared on April 23, 2010 in the journal Physical Review Letters, issue 104. The research was undertaken within the framework of the special research area TR7 “Gravitational Wave Astronomy” and funded by the German Research Association. The article “Realization of a monolithic high-reflectivity cavity mirror from a single silicon crystal” by F. Brückner, D. Friedrich et al. can be found at: http://prl.aps.org/abstract/PRL/v104/i16/e163903.
Background information
The novel technique of surface treatment can, in principle, also be applied to other crystals used in optics and also functions with visible light when the corresponding structural parameters are selected. In the present case, the scientists chose silicon and infrared laser light with a wavelength of 1,550 nm because these parameters represent good candidates for future interferometers in earth-bound gravitational wave detectors. Much of the technology used today in gravitational wave detectors was developed at the GEO600 Gravitational Wave Detector in Ruthe near Hannover. The nanostructured silicon mirror was created at the Friedrich Schiller University of Jena. Its design and characterization was undertaken in close collaboration with the Hannover Work Groups involved with GEO600. The success of this collaboration once again demonstrates the role of GEO600 as a unique think tank for international gravitational wave research.
The testing of the silicon mirror
The next step is to prove that thermal noise can actually be adequately absorbed by the specially treated crystal surface. In order to test this, the scientists will soon be developing a new silicon mirror that will be incorporated into a highly sensitive, 10-meter interferometer that is operated at the University of Glasgow. Measurements should show that the interferometer sensitivity is increased through use of the new mirrors. If this proves successful, the new technology will also be employed in the large, heavy mirrors (weighing several kilograms) utilized in the GEO600 Gravitational Wave Detector. The new technology can also be harnessed for "mirroring" tiny oscillating crystals. Through the use of cold temperatures it is hoped that Brownian motion could then be reduced to such an extent that it would be possible to directly observe the quantum mechanical aberration of the vibrating crystal described by Heisenberg's uncertainty principle. In addition to applications of the novel optics in basic research, these mirrors are also interesting for the control of high-performance lasers in laser material processing.
Special Research Area Transregio 7 - “Gravitational Wave Astronomy: Methods - Sources - Observation”
In 2002 the Deutsche Forschungsgemeinschaft established the Special Research Area/ Transregio 7 (SFB/TR 7) for gravitational wave research. The following institutions are taking part in SFB/TR 7: the Max Planck Institute for Gravitational Physics (Albert Einstein Institute) in Golm and Hannover, the Max Planck Institute for Astrophysics in Garching, the Leibniz Universität Hannover, the Friedrich- Schiller-Universität Jena and the Eberhard Karls University of Tübingen. The SFB/TR 7 is devoted to theoretical and experimental astrophysics in the field of gravitational wave research. In the investigation of the field equations of gravitation, the development of new mathematical methods stands at the forefront. The aim is to investigate the structure and dynamics of compact astrophysical objects such as neutron stars, black holes, binary systems and collapsing matter, thereby calculating their emission of gravitational waves.
In the experimental field, the design, production and application of effective reflection optics for beam splitting and beam superposition in various types of interferometers are to be investigated on the basis of diffractive structures that have been applied to highly reflective layer systems using micro- and nanostructure techniques. Complementary to this is creating the nanostructure of the crystal surfaces themselves, whose optical properties are comparable to the layer systems. The use of new interferometer topologies (signal recycling, resonant sideband extraction, active vibration insulation, cooling, QND techniques, optimized mirror systems) will significantly increase the possibility of influencing the sensitivity curve of gravitational wave detectors of the second and third generations.